Leveraging Backgrounded Membrane Imaging for Rapid and Efficient Protein Aggregation Analysis in Microbubble Aeration Experiments
In the rapidly evolving world of drug development, staying ahead of the curve demands innovative techniques. Halo Labs has tackled this challenge head-on using Backgrounded Membrane Imaging (BMI), a high-contrast imaging technique featured on Aura® PTx that can be applied to novel methods to easily draw informed conclusions swiftly and efficiently.
A recent study published in Pharmaceutical Biotechnology, “A Rapid, Small-Volume Approach to Evaluate Protein Aggregation at Air-Water Interfaces,” highlighted a unique application of BMI in which researchers utilized its high-throughput, low-volume capability to evaluate protein aggregation in microbubble aeration experiments. These experiments mimicked manufacturing processes with multiple air-water interfaces – a common cause of protein aggregation.1
During the study, samples of 100–600 µL were loaded onto membrane plate wells depending on the particle count in the samples. This feature makes BMI particularly beneficial for dilute samples, such as those used in microbubble aeration experiments where discrete bubbles are formed and detached. Unlike flow imaging techniques, such as FlowCam® or MFI™, that only analyze a portion of the sample volume due to constraints such as priming and camera frame rate, BMI filters the entire sample through one or more membrane wells, capturing all particles and providing a comprehensive analysis of the sample volume.1
Advantages of Backgrounded Membrane Imaging in Protein Aggregation Analysis
A significant benefit of Backgrounded Membrane Imaging is its ability to image particles in a dry environment on the membrane plate well. This approach effectively removes air bubbles that could otherwise be counted as particles, a common issue when analyzing liquid samples using flow imaging microscopy and light obscuration techniques.1
“While flow imaging techniques are established methods in the field, they often require sample volumes on the order of hundreds of microliters per run due to the purging and dead volume associated with flow-cell-based systems. Additionally, the finite frame rates of the detection cameras in flow imaging techniques result in the analysis of less than 100% of the sample volume injected.
As a result, flow imaging is not optimal for samples when the number of particles contained in a statistically averaged fraction of the total volume may not accurately represent the total number of particles in the entire volume— i.e., at low particle counts. BMI can analyze the entire sample volume filtered through the membrane well and is particularly useful for ‘low stress’ conditions that generate a small number of particles per unit volume.”1
A Winning Combination: Microbubble Aeration and Backgrounded Membrane Imaging
When coupled with a technique for quantifying particles at low levels (such as BMI), microbubble aeration is an effective method for accelerated surface-mediated aggregation studies. The labor and material requirements are significantly reduced from methods previously used in the literature, which is beneficial in early-stage pharmaceutical development when sample quantities may be limited. The amount of interface turnover as well as the lifetime of the interfaces between creation and destruction are also significantly reduced from previous methods. As a result, microbubble aeration may be uniquely suited for probing adsorption and aggregation processes that occur when an air interface is quickly created and destroyed to distinguish it from slower processes observed when the interface persists for longer.1
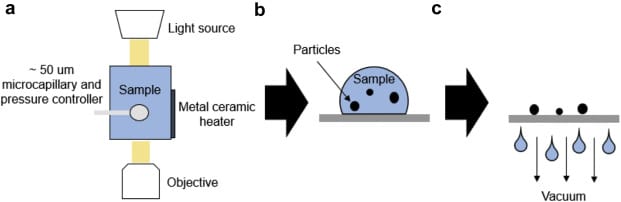
Moreover, both microbubble aeration and BMI are relatively high-throughput techniques. Traditional flow imaging techniques often require frequent washing and priming to prevent contamination from particles remaining from previous samples, making them relatively low throughput. With BMI, the entire sample volume from microbubble aeration was analyzed on a 96-well grid in less than 2 hours.1
By harnessing the combined power of BMI and microbubble aeration, evaluating protein aggregation effectively and accurately has never been easier on Aura PTx. This innovative approach not only improves the accuracy and speed of analysis but also reduces labor and material requirements, making it a promising advancement. At Halo Labs, we’re committed to pioneering novel applications of BMI, revolutionizing particle analysis, and driving us one step closer to more efficient drug development.
Related
- Light Obscuration May Not Be Enough to Detect SVPS in Your Drug Product
- Did you know you can do immunoassays on Aura®?
- Check out our App Note on protein aggregation identification and quantitation
References
1. Wood, C. V., Razinkov, V. I., Qi, W., Furst, E. M., & Roberts, C. J. (2021). A Rapid, Small-Volume Approach to Evaluate Protein Aggregation at Air-Water Interfaces. Journal of pharmaceutical sciences, 110(3), 1083–1092. https://doi.org/10.1016/j.xphs.2020.11.024